Rekan-rekan IAGI,
FOSI (Forum Sedimentologiwan Indonesia), komisi sedimentologi IAGI sedang mempersiapkan majalah Berita Sedimentologi yang rencananya akan terbit Februari 2011. Kami memilih topic geografis, untuk mempermudah persiapan majalah tsb. Rencana penerbitan 3 kali setahun:
Februari 2011: topic Sumatra, deadline for abstract submission 10
December 2010, deadline for paper submission 10 January 2011
Juni 2011: topic Kalimantan, deadline for abstract submission 10 April
2011, deadline for paper submission 10 May 2011
Oktober 2011: topic Papua, deadline for abstract submission 10 August
2011, deadline for paper submission 10 September 2011
Berita Sedimentologi akan terbit online dalam bahasa Ingris dan dalam
bentuk PDF file. Setiap terbitan kami mempersiapkan kolom tetap:
- Geo-seismic (berhubungan dengan seismic interpretation/geophysics)
- Field-work (berhubungan dengan outcrop dan pemetaan lapangan)
- Campus news (meliput kegiatan universitas yang berhubungan dengan sedimentologi)
- Student section (memberikan kesempatan kepada para mahasiswa untuk menulis extended abstract mengenai penelitian lapangan mereka /thesis)
- News (berita mengenai kegiatan-kegiatan yang berhubungan
dengan sedimentologi)
Jika anda:
1.Ingin bergabung dengan FOSI: silahkan mendaftarkan diri melalui Linked-in (www.linkedin.com): FOSI group.
2.ingin menyumbangkan tulisan:
a.silahkan menghubungi saya melalui japri
b.lihat deadline schedule di atas.
c.dengan persetujuan editorial team dan melaluis proses seleksi,
kami menerima paper yang pernah diterbitkan di bulletin lain.
d.Tulisan disiapkan dalam bahasa Ingris, gambar sebaiknya
berwarna, tapi kami menerima gambar hitam-putih.
Salam
Herman Darman
Shell International Exploration and Production B.V.
Correspondance: P.O. Box 162, 2501 AN The Hague - NL
Office: Carel van Bylandtlaan 5, 2596 HP The Hague - NL
Jumat, 14 Januari 2011
Rabu, 12 Januari 2011
A New Twist on Mud Deposition - Mud Ripples in Experiment and Rock Record
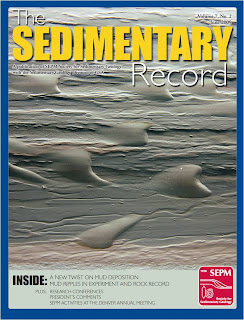
Jüergen Schieber and Zalmai Yawar
Department of Geological Sciences, Indiana University, Bloomington, IN 47405
ABSTRACT
Recent flume experiments with muddy suspensions have demonstrated that low-energy settings are not a prerequisite for the accumulation of muds and shales.Flocculation produces deposition-prone aggregates even in freshwater, and these floccules form bedload ripples at flow velocities that would also produce ripples in sandy sediments. Floccule ripples are crosslaminated but have a much higher water content (80-90 % by volume) than sand ripples and are thus subject to substantial compaction. Because of this, we must expect that original crosslaminae become severely flattened and are difficult to recognize in the rock record. Nonetheless, a survey of ancient shales has shown that certain intrinsic features of cross lamination, such as basal downlap and top truncation of laminae, are still recognizable and allow identification of current-deposited muds. The example of the Cambrian Eau Claire Formation is used to illustrate how this approach can change our perception of the depositional setting of certain mudstone successions.
INTRODUCTION
The traditional axiom on the deposition of mud, promulgated in numerous contemporary text books, asserts that deposition of mud primarily occurs in environments with weak currents. Yet, some observations have long indicated the possibility of mud
accumulation in more energetic settings (e.g., Rine & Ginsburg 1986; Walker & Harms 1971; Schieber 1998), and suggest that the longevity of this axiom owes more to a lack of data than to a preponderance of fact. Flume experiments on the deposition of mud and clay have now demonstrated that quiescent conditions are not a prerequisite of mud accumulation. Swift moving (15-30 cm/s) muddy suspensions are prone to flocculate over a wide range of salinities and sediment compositions, and the flocculated material travels as bedload in the form of floccule ripples (Fig. 1; Schieber et al. 2007). In these experiments step-wise reduction of flow velocity results in successive re-equilibration of the flow towards a new dynamic balance between flocculated clay in bedload and clay remaining in suspension. At some point a velocity is reached below which a linear decline of suspended sediment concentration occurs until the bulk of particulates has settled out of the flow. This velocity is termed the critical velocity of sedimentation (Schieber et al., 2007), and its value increases with the suspended sediment concentration of the flow.Given sufficient sediment supply, floccule ripples accrete into continuous mud beds.
FLUME STUDIES
We visualized ripples by shining strong lights through the turbid and milky looking clay suspensions, and then observing and recording from below their projections on the transparent flume bottom (Fig. 1A). In this way we were able to document the movement of flocculated clays in association with boundary layer streaks and as ripples. We also succeeded to directly observe sediment transport across floccule ripples and how sediment accreted on the lee sides of ripples (Schieber and Southard, 2009). A large portion of the bedload floccule freight is moved over the
stoss-side of the ripples in boundary layer streaks, temporarily accumulates on the crest, and then avalanches down the lee-side once enough sediment has accumulated (Fig. 2).
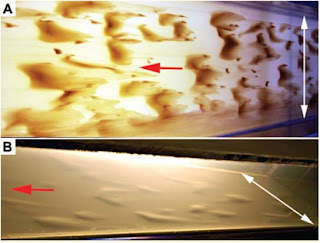
Figure 1. Viewing floccule ripples in the flume channel. (A) Floccule ripples as seen through the flume bottom with backlighting, they are migrating right to left. (B)Oblique side-view of ripples (same experiment as in A). Ripples are draped by a thin blanket of residual suspended clay that settled after the flow was stopped. Red arrowsindicate flow direction, white double arrows indicate width of channel (25 cm).
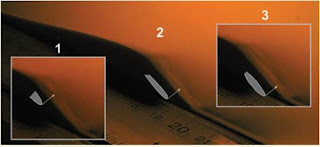
Figure 2. Oblique view of a floccule ripple that became attached to the flume wall during the experiment. Inserts show a time series of the foreset just a few seconds before (time marker 1) and after (time marker 3) the main image (time marker 2)was taken. The image sequence shows the movement of a sediment avalanche (i.e.,a flow of clay floccules) down the foreset slope. The shape of the flow is shown as a gray drawing that is offset from the actual lobe in each image, with the arrow pointing to base of the avalanche. Note the downslope movement of avalanche base between images 1, 2, and 3. The cross-section of the ripple is quite comparable to that of sand ripples. The ruler has centimeter subdivisions.
Lee-side sediment movement occurs in the form of multiple and overlapping sediment lobes that over time build up composite foresets. The gravity-driven sediment lobes spread out at the toe of the slope and form rounded lobes that we are able to see through the flume bottom (Schieber et al., 2007).
Migration rates of floccule ripples vary non-linearly with flow velocity. They range from ~20 cm/hour at a flow velocity of 15 cm/s, increasing by a factor of three to ~60 cm/hour with a doubling of flow velocity at 30 cm/s (all at 5 cm flow depth). The
latter rates are still approximately 3 to 4 times slower than those of comparable sand ripples because of the fundamentally cohesive nature of floccule ripples. As they migrate, floccule ripples have a cross-section that is quite similar to that of sandy ripples (Fig. 2) and show downcurrent dipping cross-laminae. In uncompacted clay beds these cross laminae are readily observed, and we have also visualized lateral accretion through injection of pigment pulses during experiments
(Fig. 3A). In plan view the ripple cross-lamination is manifest via rib and furrow structure (Fig. 3B). Fundamentally, these experiments indicate that many long-held
assumptions about mud deposition and mudstones do not reflect physical realities. One may wonder of course how much this helps us to interpret the rock record. Because floccule ripples initially consist to between 80 and 90 percent by volume of water, the originally inclined cross-laminae are largely oriented parallel to
bedding after compaction. Also, because these ripples are spaced 10 or more centimeters apart, in a thin section what once was a crosslaminated mud, would most likely be described as parallel laminated. So, where do we go from here?
Fortunately, there are some intrinsic features associated with cross-lamination that should be detectable through careful examination of rocks.
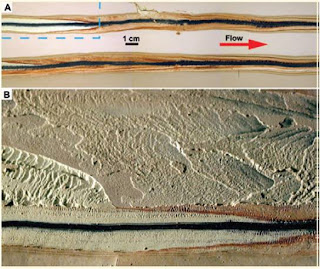
Figure 3. (A) Lateral accretion visualized by injection of pigments into flow. The claybed was scraped parallel to flow in order to expose internal layering. Pigment markersoutline clinoforms inclined to the right. Area marked with blue dashed line indicatesthe location of image (B)(B) Obliquely illuminated view of a portion of the mud bed(marked by blue dashed line in A), showing what the area just above the upper scrapelooks like once the top portion of the bed has been peeled off. Peeling exposes two setsof internal cross-laminae, a lower one consisting of white clays, and an upper one thatstarts at the pigment marker and has a reddish hue. This type of structure is identical to "rib and furrow" structure as described from ripple cross-laminated sandy deposits.
For example, in the case of a large enough specimen one may find laminae that downlap onto a basal surface or may show updip truncation. We looked for these features in a variety of ancient shale
successions, and were able to identify them in rocks from a wide range of ages. We show here some particularly striking examples from the Cambrian Eau Claire Formation in the subsurface of Indiana that were found in the course of Zalmai Yawar's Masters thesis research.
ROCK RECORD
The Upper Cambrian Eau Claire Formation of Indiana consists of fine to very fine-grained quartzitic sandstone that is interbedded with variably colored shales(Ostrom, 1970).Intervals of very fine to fine dolomitic sandstone and siltstones with glauconite are also common. The Eau Claire shales are typically dark gray, but in places they are also green, maroon and micaceous. In the studied cores the Eau Claire Formation overlies the Mt. Simon Sandstone and is in turn overlain by theGalesville Sandstone in north-western Indiana, and by the Davis formation in
north-eastern Indiana. The thickness of the Eau Claire ranges from about 120 m in
north eastern Indiana to more than 300 m in the south western parts of the statWire line logs of the Eau Claire show considerable variability from county to county, reflective of strong lateral variability of lithofacies as seen in cores. The examined portion of the Eau Claire Formation is a storm-dominated epicontinental shelf succession (Wasineeet al., 2008).
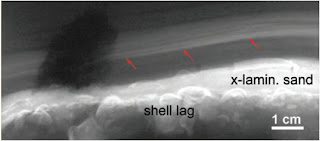
Figure 4. X-radiograph negative of modern shelf muds from the Gulf of Papua (Martin et al., 2008). Image shows a bundle of silty laminae in the base of a presumed fluid mud deposit (red arrows at base) that are inclined at a low angle to the left, suggestingcurrent transport, possibly in the form of floccule ripples. The deposit is very similar toexamples from the Cambrian Eau Claire Formation (Figs. 5, 6).
Sandstone-dominated intervals show well developed hummocky cross-stratification (HCS) in thicker beds(5-20 cm thick), and wave-ripple crosslamination, grading, and graded rhythmites in thinner beds. Shale-dominated intervals show thin, sharp-based sandstone beds withwave-ripple cross-lamination, grading, and parallel lamination on the coarse end of the grain-size spectrum. Shale beds with fine silt laminae, graded silt-clay couplets, mudblankets, and variable bioturbation are typical for the fine portion of the grain-size spectrum. Similar sedimentary structures are also found in modern storm-dominated shelf muds (Reineck et al.,1967;Reineck and Singh,1972;Aigner and Reineck,1982; Johnson and Baldwin, 1996).Aigner and Reineck (1982) suggestedthat in the case of the North Sea, windinduced gradient currents were the cause for offshore transport of sand during storms. With regard to mud-rich deposits, they followed earlier interpretations and ascribed them to deposition from stormwavedriven suspension clouds(Reineck et al.,1967;Gadow and Reineck,1969;Reineck and Singh,1972). However, careful re-examination of the original plates
of early North Sea work (e.g.,Reineck et al.,1967; Gadow and Reineck,1969)
suggests that there are low angle inclined laminae in these muddy storm deposits,
and that simple settling from suspension does not fully explain the preserved
sediments
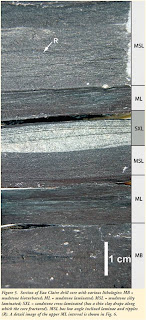
Figure 5. Section of Eau Claire drill core with various lithologies: MB =mudstone bioturbated; ML = mudstone laminated; MSL = mudstone siltylaminated; SXL = sandstone cross-laminated (has a thin clay drape alongwhich the core fractured). MSL has low angle inclined laminae and ripples(R). A detail image of the upper ML interval is shown in Fig. 6.
More recent studies of modern shelf-mud deposits suggest that wave-supported mud
suspension, in combination with tidal currents and/or fluvial discharge, may lead to
cross-shelf transport in the form of fluid mud beds (e.g.Harris et al.,2005;Palinkas et al.,2006;Martin et al.,2008). Although in these studies it is presumed that mud transport is accomplished when fluid mud layers follow gravity, X-radiographs of the sediments in question tend to show interlaminated sand/silt/mud in the basal portion of the resulting beds. Most interestingly, in a number of published examples these laminae downlap onto the basal surface (Fig. 4). In the context of our experiments this could be interpreted as resulting from bedload transport and deposition of a mixture of sand, silt, and clay. This opens up the interesting possibility that purported fluid mud deposits (e.g. Wright and Friedrichs,2006) actually consist of a lower crosslaminated portion ("invisible" if there is no sand or silt) that accumulated like the muds in our flume experiments, and have an upper,massive appearing portion that settled subsequently from a dense muddy suspension. Flume experiments set up to test this scenario are feasible and should prove informative.Close examination of the Eau Claire Formation shale beds (Fig. 5) also suggests that its shelf muds most likely did not accumulate via simple suspension settling.
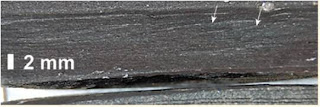
Figure 6. Close-up of the uppermost ML interval from Fig. 5, showing low angle inclined silty laminae (arrows) within a mudstone matrix. These silt-rich laminae act as surface markers in the same way as the pigment markers in our experiments (Fig. 3),and reveal lateral sediment accretion and bedload transport.
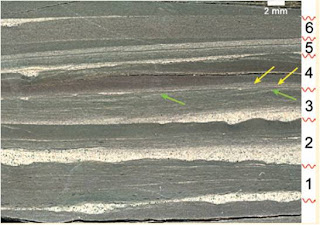
Figure 7. A succession of graded silt/mud couplets in the Eau Claire Formation.They are numbered successively and are separated by erosion surfaces. Couplet 3 shows the same inclined silty laminae (green arrows) as shown in Fig. 6, and couplet 4 shows downlapping silty laminae (yellow arrows) in its base. Couplet 5 shows silty laminae that are essentially horizontal, and couplets 1 and 2 show silty laminae that are very gently inclined. Couplet 6 has a mere hint of silt in the base, but is nonetheless most likely erosive.
The laminated mudstone intervals (ML), characterized by thin silty laminae in a
mudstone matrix (Figs. 5, 6, 7), show exactly the kinds of features that our flume
experiments predict for muds emplaced by bedload transport. For example, in Figure 6,
we see that the uppermost ML interval has inclined internal laminae that suggest lateral accretion of sediment due to bedload transport. Thus, this mud layer appears to have been deposited by currents rather than settling from suspension. Figure 7 shows multiple layers of this type and their variability. Some layers show clearly inclined silty laminae, updip truncations (couplet 3) and basal downlap (couplet 4), whereas others show indistinctly inclined or parallel laminae. Although individual layers differ from each other, their overall appearance strongly suggests a common origin. The difference in the inclination of laminae most likely reflects variations in flow direction, not a difference in depositional process. We know this quite well from field work with sandstones, where cross-bedding is clearly displayed in exposures parallel to flow, and barely visible in exposures perpendicular to flow.
Another factor that may affect "visibility" of inclined mud laminae may be the availability of contrast-producing grains. In our flume experiments, where pure clay was used, cross-lamination was only visible because we used pigment injections to mark contemporaneous surfaces. Without this the resulting clay beds would have a uniformappearance, not unlike couplet 6 and the upper portion of couplets 4 and 5 shown in Figure 7. These observations from the Eau Claire Formation suggest that a substantial proportion of the shelf muds in that particular succession did not simply
accumulate from material that settled out of suspension after storms stirred up the seabed. Instead, the available sedimentary features suggest distinct depositional events that were accompanied by initial erosion. In the context of our flume
experiments, erosion seems to have been followed by transport of flocculated mud in bedload. In view of an overall storm-dominated Eau Claire shelf, these mud beds (Fig. 7) are probably best interpreted as deposited from offshore flowing gradient currents (Aigner and Reineck, 1982). These bottom currents were most likely turbid and transported flocculated mud as bedload. Given the presence of HCS beds within this succession, the cross-laminated mudstones may represent storms of lesser magnitude that were not capable of transporting large quantities of sand, or alternatively may be the distal tails of storminduced currents that originated at a greater distance. The abundance of these muddy event beds in the Eau Claire suggests that, contrary to conventional wisdom, a substantial portion of its mudstones was deposited by currents.
CONCLUSIONS
Flume experiments are providing new insights that are transforming mudstone sedimentology. Already, basic experiments on mud deposition demonstrate that muds can deposit under energetic conditions. The associated sedimentary
structures, albeit subtle and easily missed, are preserved and allow detection of current deposited muds in the rock record. With continued experimental work we will be able to connect an increasing number of sedimentary features in mudstones to definable physical processes, allowing us greatly improved interpretations of their depositional setting. Observations made on ancient mudstones over the past decades likewise suggest that the deposition of mud is much more dynamic and complex than commonly appreciated (e.g. Potter et al., 2005). It is likely therefore, that in the
light of new insights from experiments, new opportunities will arise to derive much better information about the geologic past from ancient mudstone successions.
Langganan:
Postingan (Atom)